INTRODUCTION
In recent years, basic and clinical research on the therapeutic potential of stem cells, particularly adult stem cells, has virtually revolutionized medicine. Some types of adult stem cells have been found to have an ability greater than previously thought to differentiate into cells of different lineages, under certain conditions.[1] Regenerative cell therapy, the new modality employing these hematopoietic stem cells (HSC) for regenerative purposes, is of great interest to the scientific community—as once were its predecessors, blood transfusion and bone marrow transplantation, now routine procedures of recognized value.[1]
Bone marrow contains not only HSCs, but also other types of cells, including endothelial progenitor cells (EPC) from the embryonic hemangioblast and mesenchymal stem cells—the latter gaining clinical significance in recent years due to their therapeutic potential. Lately, cells with pluripotent capacity, called very small embryonic-like cells, have also been described. Mononuclear cells (MNC) derived from bone marrow constitute a sort of “cocktail” of different adult stem cells, among them endothelial cells, which has facilitated their extensive use in diseases with an ischemic component.[1]
One such condition is chronic lower limb ischemia, which results from a slow, progressive decrease in blood flow that reduces oxygen supply to the leg muscles during exercise.[2] Development of collateral circulation allows tissue ischemia to remain silent until vessel lumen obstruction exceeds 70%, at which time the cardinal symptom appears: intermittent claudication, muscle pain on walking that disappears at rest.[3] Disease progression is slow; after 5 to 10 years of followup, more than 70% of the patients show no changes in symptoms, while 20 to 30% have worsening symptoms requiring intervention.[4,5]
Prevalence of chronic lower limb ischemia based on physical examination ranges between 5 and 10% in the population aged 55–65 years and between 10 and 15% in those aged >65 years. When hemodynamic tests are performed, prevalence rises to 20–25% in patients aged 55–65 years and over 30% in those aged >65 years.[6] Prevalence at necropsy data is 30–40% in those aged 55–65 years and over 60% in those aged >65 years.[7] Incidence of the most severe form, critical ischemia, has been estimated at 500 cases per million population per year worldwide, [8] which would correspond to 6000 new cases annually in Cuba’s population of approximately 12 million.
Recent technological developments and use of advanced experimental animal models have led to new strategies to treat ischemia, especially in the legs. Some have already shown promising results in clinical trials.[9,10]
The body’s ability to spontaneously develop collateral circulation is an important positive factor in response to occlusive vascular disease and decisively affects severity of residual tissue ischemia. Neoangiogenesis (new vessel formation) and vasculogenesis (formation of new vessels from pre-existing ones) may be sufficient to preserve muscle tissue integrity and function, and so have been the basis for developing new treatment approaches.[11,12]
Angiogenesis is a dynamic process of endothelial cell proliferation and differentiation in which new functional vascularization results from the coordinated activity of endothelial cells, extracellular matrix and surrounding cells. Vascular growth factors are among the major physiological stimuli of angiogenesis; these include the prototype vascular endothelial growth factor and others, such as angiopoietins 1 and 2 (Ang-1, Ang-2), transforming growth factor-ß and basic fibroblastic growth factor. Some of these angiogenic factors, mainly vascular endothelial growth factor and fibroblastic growth factor, have already been used in human clinical trials for ischemic cardiomyopathy and lower limb ischemia.[13]
These trials have generally used only one growth factor, a limitation when studying a process that requires interaction among several elements, EPCs among them—hence, the suggestion that future trials employ combinations of different angiogenesis growth factors. However, such procedures are more complex and expensive, requiring highly specialized laboratories.[14]
One alternative is to provide cells that can influence growth factor release or EPCs that can directly affect angiogenesis.[15] It has recently been shown that adult bone marrow is a rich reservoir of several types of EPCs and HSCs that can contribute to vascularization, both during the physiological embryonic period and postnatally.[16] Due to its potential angiogenic effect, autologous implantation of bone marrow-derived mononuclear stem cells is being implemented to good effect in patients with severe ischemic complications, in what has been described as “a revolution in regenerative medicine.”[17,18]
The advantage of cell therapy to induce angiogenesis is that it could promote endothelial regeneration and release of several angiogenic growth factors. Furthermore, the existence has been established of cell subpopulations in bone marrow that can differentiate into myogenic and vascular cells (angiomyogenesis).[17] Autologous bone marrow MNC transplantation has been proposed as a safe and effective angiogenic therapy, because of these cells’ natural property of yielding EPCs and several angiogenic factors and cytokines.[19]
To date, there is scant literature on prognostic factors for autologous HSC transplantation for ischemic conditions; nor is there much on associated adverse effects. There are conflicting results regarding its true benefit, and it has been observed that individual patient characteristics decisively influence outcomes. A REPAIR-AMI study analysis subgroup found larger infarcted area and left ventricular dysfunction to be associated with greater autotransplantation success rates.[20] The BOOST study concluded that transplant success did not depend on patient age, nor degree of dysfunction, nor therapy timing but rather was influenced by presence of diabetes and other chronic conditions;[21,22] experimental studies have also demonstrated that diabetes and other systemic diseases significantly reduce HSC angiogenic potential.[23]
The conclusion from all this? Stem cell therapy can be beneficial, but not for all patients. Hence the importance of identifying patients who are most likely to benefit. Identification of prognostic factors could help determine the most appropriate candidates, avoiding raising false expectations in those patients who would not benefit. This would also be a contribution to health systems’ rational use of human and financial resources, both substantially implicated in this form of treatment.
There have been no Cuban studies of possible factors associated with poor angiogenic response after autologous HSC transplantation, an indisputably important question for deciding which patients are best candidates for this therapy. Our objective, then, was to identify clinical and laboratory factors that could suggest poor prognosis for angiogenesis after HSC autograft in patients with chronic lower limb ischemia and to establish a preliminary scale to stratify patients by risk.
METHODS
Study type and population A retrospective analytical study was carried out of a case series of 47 adult patients with lower limb ischemia who underwent autologous HSC transplantation in the Dr Gustavo Aldereguía Lima University General Hospital in Cienfuegos, Cuba, between January 2007 and December 2010. Patients with incomplete clinical or laboratory records were excluded.
Data source Data on variables selected were taken entirely from clinical records of patient care from prior to autotransplantation through follow up.
Study variables Independent clinical and laboratory variables assessed are summarized in Table 1. The dependent variable, successful angiogenesis, was defined by avoidance of amputation for one year post-transplantation.
Table 1: Study variables
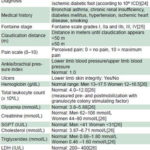
ESR: erythrocyte sedimentation rate LDH: Lactic dehydrogenase SGPT: serum glutamic piruvic transaminase
Measurement of ankle/brachial pressure Blood pressure was measured prior to HSC implantation and 4, 8 and 16 weeks postimplantation. The ankle-brachial pressure index was calculated by dividing lower limb systolic pressure (attained by Doppler ultrasonic flow meter) by systolic arm pressure (measured by conventional auscultation) with the patient lying supine.[27] Posterior tibial and lower dorsal pedal artery pressures lower than brachial pressures indicated important arterial obstruction. In borderline cases, measurements were taken before and after exercise.
MNC separation method The procedure used by Hernández[1] was followed to mobilize peripheral blood MNCs: patients were given 10 µg/kg of domestically-manufactured recombinant human granulocyte colony stimulating factor (LeukoCIM, CIMAB SA, Cuba) subcutaneously every 12 hours for a total of 4 doses. Three hours after the last injection, 800–1000 mL of whole blood were extracted, amount depending on the patient’s clinical condition. MNCs were then concentrated by sedimentation after adding 1 mL of 6% hydroxyethyl starch per 6 mL whole blood.
MNC and HSC count and assessment of leukocyte viability To evaluate viability and cell count, Hernández’s method[1] was used, with slight modifications dictated by resource limitations in our institution: a small fraction of cell suspension was used for cell count, viability testing by trypan blue exclusion and microbiology. Cell count pre- and postmobilization was performed in a Neubauer chamber, since an automatic cell counter was not available. All readings were done by the same observer to avoid interobserver error, the main limitation of this method. To reduce intraobserver error, duplicate readings of each sample were performed and averaged. Differential cell count was carried out by optical microscopy of cell concentrate stained with May-Grünwald Giemsa. Lymphocytes, monocytes, lymphocytoid and monocytoid cells, immature myeloid cells, proerythroblasts and erythroblasts were considered MNCs. MNC concentration was not available in all patient records so not used in prognostic analysis. Mean MNC concentration for 34 patients was 2.5 x 109/L.
MNC implantation MNC were injected intramuscularly in the gastrocnemius and quadriceps muscles, per Hernández.[1]
Data processing Percentages were calculated for general variables: sex, medical history and Fontaine stages;[25] mean and standard deviation were calculated for age. Results were presented in frequency distribution tables. Logistic regression analysis was used to study categorical variables representing possible prognostic factors for deficient angiogenesis. In univariate logistic regression, variables showing a coefficient of ≤0.1 (p ≤0.10) were considered prognostic factors, due to small sample size. Values from one to four were assigned to variables when their p values corresponded to 0.10, 0.05, 0.025, and 0.01 respectively; these values were cumulated to create a preliminary scale, modeled on the approach for MNC autograft in the heart,[28] the only one found in the literature for similar purposes. The significance level was set at p ≤0.05. Data were processed using SPSS 15.0 Windows statistics.
Ethical considerations The study relied exclusively upon administrative data, taking measures to ensure patient anonymity. It was approved by the ethics committee of Dr Gustavo Aldereguía Lima University General Hospital.
RESULTS
Mean patient age was 64 years; male sex predominated (59.6 %). Hypertension (HT) and diabetes were concurrent in 23.4% (11); a further 17% (8) had both these conditions plus ischemia cardiomyopathy; and 17% reported smoking. Fontaine stage IV predominated, with 53.2% (25) (Table 2).
Regression analysis of clinical parameters revealed age ≥60 years and a pain scale score of 10 to be significant, resulting in prognostic scores of 3 and 2 points, respectively (Table 3).
Regression analysis of laboratory values found final leukocyte count of <20 x 109/L and glycemia of >6 mmol/L to be significant, for prognostic scores of 4 and 2, respectively. The final variable to enter the scale was triglyceride level, with a prognostic score of 1 (Table 4).
Thus, five variables were identified as potential predictors of poor prognosis for angiogenesis in autotransplanted patients: postmobilization leukocyte count of <20 x 109/L, age ≥60 years, pain scale score 10, glycemia of ≥6 mmol/L and triglyceride level of >1.8 mmol. Assigned risk scores were 4, 3, 2, 2 and 1, respectively, creating a potential scale of 0–12 for predicting risk of amputation because of poor angiogenesis.
Table 2: Patient characteristics (n=47)
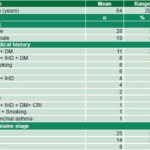
IHD: Ischemic heart disease DM: Diabetes mellitus HT: Hypertension CRI: Chronic renal insufficiency NA: Nonsmoker with none of listed diseases
Table 3: Regression analysis and risk scores of clinical variables
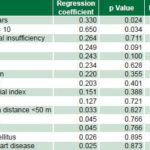
Table 4: Regression analysis and risk scores, laboratory variables
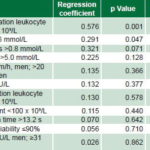
ESR: erythrocyte sedimentation rate LDH: lactic dehydrogenase SGPT: serum glutamic piruvic transaminase
Table 5: Observed angiogenic potential by predicted (n = 47)
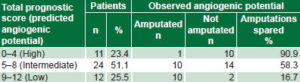
Overall, 55.3% (26/47) of patients were able to avoid amputation. Of patients with scores between 0 and 4 (high predicted angiogenic potential), 90.9% (10/11) were spared amputation. Of patients with scores between 9 and 12 (low predicted angiogenic potential), 16.7%, (2/12) were spared amputation and 58.3% (14/24) of those in the mid range of predicted angiogenic potential (Table 5).
DISCUSSION
Clinical application of hematopoietic stem cells in diseases with an ischemic component, rapidly extending in medical practice, has two essential advantages: immunologic, because rejection is not a problem; and ethical, because the patient’s own tissue is used, hence there is no risk from introduction of foreign matter. However, clinical and laboratory factors predictive of angiogenic potential and successful outcomes in individual patients have not been fully described.[29] There is no Cuban or international research on prognostic factors for autotransplantation in lower limb ischemia with which to compare our results, but clinical trials of HSC for cardiac and brain ischemia[30,31] and relevant experimental animal models do provide some context for comparison.
Aging has been implicated as an adverse prognostic factor in myocardial ischemic tissue autotransplantation, as reported by Li.[31] This is consistent with our finding that age ≥60 years constituted a prognostic factor (risk score of 3).
Peripheral arterial ischemia is the result of different diseases affecting lower limb vascularization, generating pain, incapacity and impaired quality of life. Critical ischemia produces great morbidity, mortality and chronic pain. The maximum score of 10 on the pain scale represents the most intense pain perceived by the patient, corresponding to greater vascular damage.[32–34] Since HSCs need a minimum of circulation for nourishment to be able to initiate neoangiogenesis, it stands to reason that greater pain intensity, an indirect indication of scant vascularization, would predict low angiogenic potential, as we found. Consistent with our results, O’Neill found neovascularization capacity potentially reduced in patients with ischemic cardiomyopathy producing pain,[35] and Fernández found reduced in vitro capacity for migration and colony formation in HSCs from patients with critical lower limb ischemia and pain.[36] In our study, pain was a moderately important predictor, with a score of 2, consistent with these and other lines of evidence.[36,37]
Adult bone marrow stem cells have many different subpopulations capable of transdifferentiation into several cell types, depending on their membrane expression markers.[38] According to their capacity for neovascularization, they can be divided into two types: CD34 positive and CD34 negative.[39] Recent experimental studies have demonstrated that CD34-positive EPCs can be mobilized into systemic circulation and can contribute to neovascularization and re-endothelialization after birth.[40,41] A decisive role in neoangiogenesis has been demonstrated for stem cell number and/or concentration.[12,42] Experimental studies in animals have shown that ischemia per se can induce stem cell mobilization to peripheral blood; particularly in mouse models, administration of granulocyte colony stimulating factor after ischemia has resulted in decreased mortality, reduced infarcted area and increased regeneration of myocytes and vascular structures.[41] However, this has not been convincingly demonstrated in clinical trials.
A larger number of MNCs mobilized to peripheral blood increases the probability that there is also a larger number of HSCs, which would improve prospects for successful neovascularization.[42] We defined this by postmobilization leukocyte count, and it resulted in the most powerful predictor of autotransplantation success, with a low count contributing four points to the scale. We therefore believe this prognostic factor to be essential for assessing likely autograft success. In fact, in the group with the best prognosis (scores 0–4), the only failure was in the single patient whose postmobilization MNC count was <20 x 109/L. Hernández found that most patients with postmobilization leukocyte counts of <20 x 109/L had low autograft success rates, and so in subsequent studies continued mobilization every 12 hours until adequate values were attained.[11] Our results reinforce the importance of continuing cell mobilization until the values are >20 x 109/L, to ensure greatest likelihood of angiogenic success in the presence of other poor prognostic factors.
One of our study limitations was that incomplete patient information meant our logistic regression did not include MNC count in peripheral blood, precisely where most HSC are found. It will be critical to include this variable in any future development of a prognostic scale.
Another limitation was the use of manual methods rather than automatic counters for absolute leukocyte count. Observer error is inherent in manual counting, although we minimized interobserver error by using only one observer and intraobserver error by averaging repeated observations on each sample. Manual and automated counting have been found to have similar equivalence coefficients (0.950 vs. 0.920).[43,44] Several authors have shown that automatic hemocytometers optimize time and laboratory resources but tend to count approximately 10 cells less than manual counters and do not discriminate cell types as well as manual counters, especially in the granulocytic and erythroid lines.[43–46] This is particularly important to keep in mind in this type of study because patients receiving granulocyte colony stimulating factor mobilize large numbers of these cells into peripheral circulation and automated counting could substantially underestimate final postmobilization counts.
Diabetes mellitus is associated with increased risk of atherosclerosis and poor prognosis following vascular occlusion,[47] as well as with disturbances in endothelial cell and monocyte maturation.[48] Neovascularization does not consist only of angiogenesis but also involves bone marrow-derived EPCs, which participate in vasculogenesis, contributing 25% of blood vessel formation.[49] The number of EPCs in circulation is relatively low but increases in response to trauma or ischemia, mobilizing from bone marrow to periphery.[50]
Some studies have shown patients at risk for coronary disease and diabetes to have reduced numbers of circulating EPCs.[51] Animal research suggests diminished vasculogenesis in diabetes, since EPC proliferation and differentiation are less in diabetic than in nondiabetic animals.[52,53] Thus, we can infer that hyperglycemia should negatively influence neovascularization, exactly what we observed in this study, where diabetes was a moderately strong prognostic factor, contributing two points to the predictive scale.
Alterations in plasma lipoproteins and lipid metabolic disorders are among the most firmly established and atherosclerosis risk factors.[54] After a usually prolonged asymptomatic phase, atherosclerosis can become manifest; one clinical expression is chronic lower limb ischemia with intermittent claudication.[55] Thus, increased blood lipids, especially triglycerides, represent a direct prognostic factor, notably increasing atherosclerosis and consequently vascular occlusion throughout the body. This reduces nourishment availability for implanted stem cells, limiting their ability to differentiate into vessel-forming endothelial cells.[56] Increased triglycerides were a minor prognostic factor for failed angiogenesis in our study, contributing one point to the predictive scale.
The main limitations of this study that may have affected its findings are those inherent in its small sample size, its local nature and lack of randomization, possibly explaining the absence of an effect of such recognized risk factors as hypercholesterolemia, chronic renal insufficiency and advanced Fontaine stage.
The literature reviewed did not identify a predictive scale specific to HSC autotransplantation for lower limb ischemia, which led us to extrapolate ours from one used for cardiac ischemia. The scale thus developed is therefore preliminary by definition, subject to all the limitations inherent in this type of study, and requires further research and modification. Nonetheless, we have provisionally identified factors that should be taken into account when deciding which patients are most likely to benefit from HSC autograft. Our study’s ultimate contribution will depend on future research to corroborate the predictive value of the possible prognostic factors found and the clinical usefulness of the risk scale developed.
CONCLUSIONS
Five clinical and laboratory variables were identified as possible predictors of poor prognosis after HSC autograft for chronic lower limb ischemia. The scale thus created has potential for classifying patients according to their high, intermediate or low angiogenic potential. Generalization of these results is subject to corroboration and refinement by further research.
ACKNOWLEDGMENTS
Thanks to Latin American Medical School students Eliana Paola Álvarez Cabral and Flavio Andrés Bovio Caltieri, for their invaluable help in data collection and to the Dr Gustavo Aldereguía Lima University General Hospital